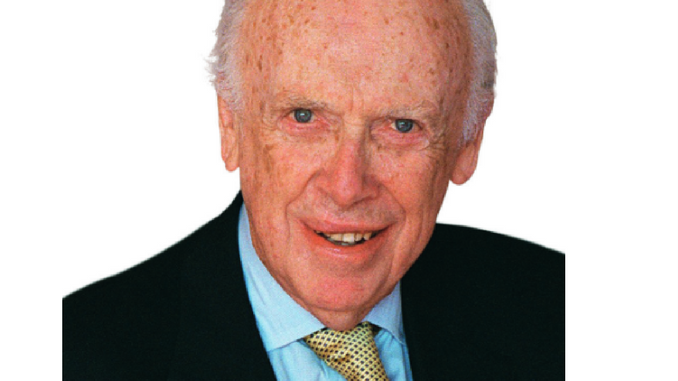
Nobel laureate Jim Watson is calling on the cancer community to take a long hard look at what has been achieved by blocking the molecular signals that drive individual cancers
Sixty years after Jim Watson and Francis Crick famously resolved the double helix structure of DNA, which opened the door to understanding the nature of cancer, there is still no cure in sight for advanced disease. Now Watson, the surviving member of the duo, who went on to pioneer and write the book on molecular biology, and led the Human Genome Project as its first director, is questioning whether genetic approaches to treating cancer can ever lead to the breakthroughs we need.
At 85 years old, Watson has spent recent years applying his vast knowledge and impressive intellect to the problem of incurable cancers, and has reached the following conclusions: To cure cancer you need to kill cancer cells. Targeted biological therapies don’t kill cancer cells, they are not curing cancer and it is unlikely that they can be made to do so in a practical or comprehensive way in the near future. It’s time for a change in strategy.
“We know the current approach is not working, because on the whole it has made no dent in cancer mortality,” he says.
Watson is speaking in his study at the Cold Spring Harbor Laboratory, New York, the research and education institution he directed and led from 1968 until 1994, and where he now holds the salaried position of chancellor emeritus. “They call me emeritus because they don’t always like what I say,” he remarks with the irreverent humour that has characterised his long career at the cutting edge of science.
But his point about targeted therapies is completely serious. He offers the example of the EGFR inhibitors Iressa (gefitinib) and Tarceva (erlotinib). “There’s no doubt EGFR inhibitors work best against cancers that contain activating mutations in their tyrosine kinase EGFRs, which is the case in about 10% of lung cancers. But even in the people they really work in, they work for about a year, tops. Then resistance. So if you look at their effect on lung cancer mortality, it really isn’t measurable, because it’s 10% of cancers, and one extra year… The cancer world has ignored resistance saying we’ll just get another drug. The problem is that once we get resistance the cancer is usually resistant to other drugs. Other drugs are not so effective, and the cancer becomes incurable.”
Watson still takes an impish delight in having a go at ‘the cancer research establishment’ – but he doesn’t want people to use that as a reason to dismiss what he is saying. He too had high hopes about the potential of targeted drugs in the early days – indeed Tarceva was co-developed (with Genentech) by a company Watson helped found at Cold Spring Harbor back in 1983, under the name Oncogene Science, later renamed OSI Pharmaceuticals. “I’m not blaming anyone,” he insists.
“We didn’t really appreciate how important it was to kill the cell – just kill it… All the good chemotherapy drugs were isolated because they caused apoptosis. Poof! The cells die. But these targeted therapies don’t kill. They stop the growth of the cell. But they don’t necessarily kill them. They can sometimes lead to apoptosis, but not in a simple clean fashion. That wasn’t appreciated.”
For years, Watson recalls, “we were all looking for the [VEGF] inhibitor that would block angiogenesis. I was part of it, and I became quite enamoured of this stuff, really because it seemed that we could get away from chemotherapy. But the irony of it is that Avastin [approved in the US in 2004] only works in conjunction with chemotherapy.” And as he points out, chemotherapy, being strongly mutagenic, tends to sow the seeds of resistance in the cancer it aims to destroy.
For the past few years, Watson has been turning his attention to things that all cancer cells have in common, however advanced the stage, no matter how chaotic and mutated the cancer cell, with a particular focus on the cancers that are the most resistant to treatment. So rather than looking for ways to inhibit the ‘always on’ signals that typically trigger particular cancers (HER2, RAS, RAF, MEK, ERK PI3K, AKT, mTOR and the rest), he is searching for weaknesses in the key regulatory and metabolic features that are common to all ‘always on’ cancer cells. He argues that we should focus far more on the wide range of metabolic and oxidative vulnerabilities that arise as a consequence of the uncontrolled growth and proliferation capacities of cancer cells.
Metformin, the type 2 diabetes treatment, offers some vital clues, he says. Known to protect against a wide variety of cancers, and to selectively kill cancer stem cells (which are normally highly resistant), it is now in trials to see whether it can augment response to cancer treatment. Watson is not confident the results will be positive, but is convinced something significant is going on which needs to be better understood. Metformin is Watson’s kind of drug – it seems to work better in cancers that are hardest to treat. “A really intriguing thing about metformin is that it kills triple receptor-negative breast cancer much better than say lobular breast cancer. So it kills the nastiest cancers, it doesn’t kill the others.”
It turns out, he says, that metformin kills cancer cells that have lost both copies of the powerful p53 tumour suppressor gene much better than those with both p53 genes in tact. “Even though normally p53 promotes apoptosis and that ability has been taken away, it still kills tumour cells.” This gives Watson reason to believe that the drug may prove to be more active against late-stage cancers where cells are so mutated that most will have lost both their p53 genes. He also cites research by Michael Pollak, head of cancer prevention at the Department of Oncology at McGill University in Montreal (Cancer Discov 2012, 2:778–790) indicating that metformin kills cells that can’t handle stress. “This means that if the cell can’t handle stress then you can kill it. So what we need to find out is if there are any drugs that will essentially inhibit our stress-handling systems. I want a pre-existing one because I don’t want to have 10 years to wait to develop one.”
“What we need to find out is if there are any drugs that will essentially inhibit our stress-handling systems”
In January this year, Watson published an article in the Royal Society journal Open Biology (vol 3, p120144), that draws together diverse evidence on something else that he believes to be a common factor playing a role across late-stage cancers. Under the title Oxidants, antioxidants and the current incurability of metastatic cancers, Watson sets out the hypothesis that, while high levels of oxidants are known to be mutagenic and dangerous, low levels of reactive oxygen species (ROS) may be essential for the proper functioning of cellular apoptosis.
This theory has obvious implications for the potential harm being done by the huge industry in anti-oxidant foods, drinks and supplements. As Watson remarks in his paper, “Blueberries best be eaten because they taste good, not because their consumption will lead to less cancer.” But it is the implications for overcoming the resistance to cancer therapy that Watson believes merit far more attention than they are currently receiving.
The hypothesis is intriguing because it has the potential to throw light on many seemingly unrelated observations, which are set out in Watson’s paper.
- The importance of ROS in the processes that induce cell death – both in the body’s normal regulatory function and in many chemotherapies
- Absence of ROS – hypoxia – as a characteristic of resistant cancer cells, including cancer stem cells
- The failure of anti-angiogenesis therapies to kill cancer cells without concomitant chemotherapy (the cells become hypoxic as their blood supply is choked off)
- The negative results of trials of vitamins A, C and E to prevent cancer, with vitamin E actually being associated with a small increased risk of many types of cancer
- The observation that cancer cells resistant to chemotherapy tend also to be resistant to radiotherapy, which implies a common mechanism of resistance.
Missing from the evidence presented in the Open Biology article is another interesting part of the jigsaw puzzle that Watson received from a reader in response to its publication, and which has convinced him more than ever that he is onto something very important. It shows that, when it comes to preventing type 2 diabetes, “exercise-induced oxidative stress ameliorates insulin resistance”, and taking anti-oxidant ‘health’ supplements can preclude the health benefits of exercise in humans (PNAS 2009, 106:8665–70).
So a picture is emerging that somehow links the metabolic condition of type 2 diabetes with cancer (and Watson suspects some degenerative diseases as well). Metformin seems to have an effect in both. We know metabolic syndromes and obesity are risk factors for cancer, we know exercise is preventive against cancer and diabetes, and now, at a biological level, this role of ROS and anti-oxidants seems to be emerging. And all of this, says Watson, has probable connections to the ‘Warburg effect’, an observation known about since the 1920s that cancer cells tend to produce energy in a way that differs from normal cells, the chief disparity being that they do not require oxygen, but use a high rate of glycolysis (up to 200 times higher than in normal cells), followed by lactic acid fermentation.
Watson is the first to concede he doesn’t have the answers, but he’s convinced he’s asking the right questions and refers to his Open Biology paper as “my most important work in years.”
“I’m feeling slightly frustrated that I can’t do something. But I stopped doing science when I was 33,” he says. While he was still boss at Cold Spring Harbor he could at least have directed some of the institution’s limited resources in this direction. But now, he concedes, “all I can do is write papers and hope that readers are open to unorthodox ideas.”
“I’m feeling slightly frustrated that I can’t do something,but I stopped doing science when I was 33”
Cold Spring Harbor Laboratory does in fact convene occasional meetings bringing together people working on metformin, for instance, but that is a far cry from the concerted research effort that Watson argues is needed. Hence his plea to those who control the bulk of cancer research funding to take an honest look at the prospects that targeted cancer therapies will ever deliver a cure for cancer, and consider whether it may not be time to change tack.
“We can carry on and sequence every piece of DNA that ever existed, but I don’t think we will find any Achilles heels. We’ve had about 10 years. It’s not the story I wanted to hear. I would have hoped for a lot more success.”
So what would Watson do if he were in charge? “My own solution is to identify people who have ideas about drugs that will attack the uniqueness of the biochemistry of cancer cells. We still don’t know the reason for the Warburg effect…. If I had two billion dollars I would give it to 20 biochemists, give them $100 million each and tell them – go to it. You have to unleash biochemistry, and there is essentially no biochemistry left because everyone moved into DNA.”
Not surprisingly, perhaps, Watson puts enormous faith in the power of unfettered intelligence, while he rarely misses an opportunity to needle the movers and shakers in cancer research who control where the big money is spent, and sit on grant committees and peer review boards. In his Open Biology article he identifies the “inherently conservative nature” of the cancer research establishment as “the biggest obstacle today to moving forward effectively towards a true war against cancer,” because they are “still too closely wedded to moving forward with cocktails of drugs targeted against the growth-promoting molecules.”
Watson himself has never sat on a peer review board, and is proud that he has never been an insider. “I generally find it does not pay to argue with the establishment or hope that they will change their mind,” he says. “Crick and I didn’t try to change the protein-oriented world. We just did our own thing and it worked.” (Many leading scientists had been expecting the secret to inheritance to be found in proteins – not least because of their diversity and potential complexity – so the discovery that it is based on sequences of only four nucleic acids was breathtaking.)
Watson’s formula for success is: “Read a lot, go to meetings, travel, try to have a job in a place where you are surrounded by bright people. You need people to talk to, but you have to get new ideas, which you only will get from reading something from another field that doesn’t seem related but is.”
Being bright doesn’t make you successful, he says. “Most geniuses are precocious because they have phenomenal memories. You can remember a lot which helps you solve problems. A chess grandmaster will have in his head 5000 games – every move. So it takes a good memory to start with. Though that won’t make you a world champion because you have to, in some sense, be wise.”
For Watson, Cold Spring Harbor Laboratory represents the sort of place he is talking about, where people can spend time surrounded by bright people and have time to talk and generate new ideas. That is what it was for him, back in 1948 and 1949, when he spent two hugely formative summers there in the company of some of the world’s best scientific brains, including Salvador Luria, who had taken Watson on as a PhD student in his lab in Indiana University, and was immersed in pioneering work on genetics in microbes that would later win him a Nobel Prize.
“In those days you didn’t spend the summer in Indiana. There was no air conditioning. People came here to the coast so they had someone to talk to. Then there was a tradition that doesn’t exist now. Summer was good for talking. And then you would do the experiments. Now people think that the summer is a time to do experiments. So the summer isn’t a relaxed period any more.”
But important though all this is to achieving scientific success, more important still, says Watson, is being in the right field at the right time. “You have to be in a field that is going to move. And that is a very hard thing sometimes to guess.”
Watson’s own track record on this score shows he’s done better than most, which is one reason why, having first become interested in curing cancer before even enrolling in his first course on tumour viruses back in 1947 – on account of a 40-year-old uncle who was dying of melanoma – he has remained a key player in propelling cancer research forward for more than 65 years.
Having started at the tender age of 15 majoring in zoology at Chicago University, with ambitions to becoming an ornithologist, Watson came across Erwin Schrödinger’s What is Life? – a book that inspired and influenced many important brains of his generation. It prompted him to switch focus to the work being done by the Indiana-based microbiologist Luria and others looking at the genetics of bacterial viruses. Picking up on the achievement of the Caltech-based chemist Linus Pauling in using X-ray crystallography to determine the physical structure of amino acid, Watson then made the correct call to learn related techniques to determine the structure of DNA, which he famously did with Francis Crick as a post doc research project at Cambridge University. Returning to the US following this epochal discovery, he took up a post at Harvard teaching tumour viruses, then switched focus to RNA, “because we had to find out how the information in DNA got into proteins.”
“I had just learnt that bacterial viruses carry enzymes involved in helping their replication, so I thought maybe the essence of cancer was a virus coded for an enzyme involved in DNA replication. And this enzyme, when incorrectly integrated in a host cell, could just be the signal to start the cell cycle.”
In 1959 Watson attended a session of the American Association for Cancer Research (AACR) on the polyoma virus – a DNA virus known to cause all sorts of tumours in immunocompromised mice. “I realised it was very small. It might be so small it would only have a few genes… This was before recombinant DNA, before we even knew about messenger RNA. It was just the idea that you could get mutants and so on. Not very precise. The idea that the true essence of tumour viruses was turning on the cell cycle. And to within limits that was right.”
Watson carried on with his work, “doing the fundamentals of molecular biology,” but kept an eye out for where the action was happening in other areas. One of these areas involved three people who were looking at the polyoma virus from a DNA perspective: Renato Dulbecco in the Salk Institute (a close collaborator with Luria, who Watson knew from his Cold Spring Harbor summers), Leo Sachs in Israel, and Michael Stoker, also known to Watson, from his Cambridge days, who was then at the Institute of Virology in Glasgow.
“So I followed what they did. In The Molecular Biology of the Gene [still a standard textbook, now in its 7th edition], I write a chapter on cancer. It’s the first time you have ever exposed the world of molecular biology to the cancer problem.” (It was nice to write because there were no referees, he adds. “I could write whatever I liked.”)
In 1968, a young biologist named Joseph Sambrook, working in Dulbecco’s lab, found evidence that the SV40 genome was integrated into transformed cells. “The basic idea was right. That’s what got Dulbecco his Nobel Prize.”
Meanwhile, a financial crisis at Cold Spring Harbor Laboratory had prompted Watson to move in to save it, and in 1968 he took over running the place full time. He made another good call, to set up the Tumor Virus Group, and hire Sambrook to head up a cancer virus group. What followed was a tremendously productive period. “The idea was to find the genes – mutants and so on,” Watson explains. “That seemed really hard. And suddenly recombinant DNA came along.”
Recombinant DNA was a game-changing genetic engineering technology invented in California, at Stanford and UCSF, which, in addition to providing a basis for the biotechnology industry, opened the way for exploring the genes of cancer cells – though in the face of strong opposition from people concerned about the implications of this new technology. “I spent four years fighting the environmentalists, who didn’t want us to do recombinant DNA,” says Watson.
It wasn’t long before the first oncogene was isolated – Ras, discovered simultaneously by Michael Wigler at the Cold Spring Harbor Laboratory, Robert Weinberg at the Massachusetts Institute of Technology (who’d previously worked in Dulbecco’s lab) and Mariano Barbacid, a Spanish molecular biologist working at the US National Cancer Institute. “And the moment you found the genes, you wanted to find the protein product and then you wanted to find the inhibitor.”
Then came the first tumour suppressor, Rb, the gene responsible for retinoblastoma, which had been known about since the 1970s, and was isolated in 1986 by Stephen Friend working in Weinberg’s MIT lab. The work showing “in a clean way” that Rb was in a tumour was done by the adenoma virus group working at Cold Spring Harbor Laboratory. Watson also successfully argued for, and then served as first director of, the Human Genome Project that first identified and mapped the entire set of 20,000–25,000 human genes.
“And now we know there are at least 10 tumour suppressors for every real driver. Most of the control is inhibitory,” says Watson, summing up what he considers to be a job well done.
Sadly, despite the high hopes, this incredible journey of discovery, which identified the genetic mutations that cause cancer, has not resulted in finding cures. Watson sees no reason to believe that carrying on endlessly sequencing tumour DNA will deliver breakthrough new treatments.
Right now, he believes that finding out more about the metabolic weakness in cancer cells is a far more productive way to proceed.
If this sounds like a recipe for spending another 20 years elucidating every dot and comma of the metabolic process of normal and cancerous cells, that is certainly not how Watson sees it. “The question of curing cancer is a practical question that is somewhat separate from the pure research of how does cancer work,” he says, and he argues that it is the people who want to carry on with the DNA sequencing who are looking to gather knowledge for its own sake.
“Propelling me 40 years ago to turn the Cold Spring Harbor Laboratory into a major site for unravelling the genetic underpinnings of cancer was the belief that once the gene-induced molecular pathways to cancer became known, medicinal chemists would go on to develop much more effective gene-targeted drugs,” Watson writes in the introduction to his Open Biology article.
He never believed in the 10- to 20-year timetable proposed by the proponents of the War on Cancer back in 1970. But he does feel we are there now. We finally know enough to turn the knowledge gained, not only about genes but also about cellular metabolism, into much more effective treatments.
“I didn’t think I had any chance when I was 25. Now I think there is a chance. Now I’ve reached 85 I think I want to reach 90. The reason is, I want to see if we can cure cancer in five years. I’m not interested in curing it in 20 years.”
“I want to see if we can cure cancer in five years, I’m not interested in curing it in 20 years”
He believes that this timetable can be met if the right people have enough resources to get the job done. “Whether we cure cancer or not is likely to depend on whether some private very rich people give money to non-traditional sources. I’d just like some rich person to give me $200 million. But who is going to give money to an 85-year-old? So going back to what I said before: two billion dollars could provide $100 million each for 20 of the best biochemists in the world.
“Some things you can do with your wits alone. You can write a text book. But you can’t produce a drug. It doesn’t matter how bright you are, you need the money. Even though it sounds like I’m criticising everyone, and it sounds like sour grapes, I just hope someone realises that what I’m proposing is actually very practical.”