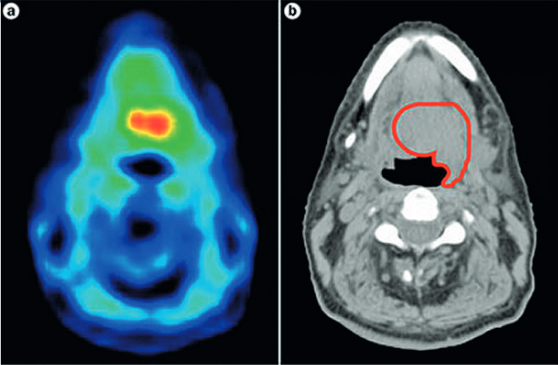
Advances in imaging and molecular biology are opening up a wealth of new avenues for research and treatment in radiotherapy. But could progress be held back by a lack of public awareness of the potential for innovation?
About half of people diagnosed with cancer in developed countries are likely to receive radiotherapy as part of their treatment, so improving the safety and efficacy of radiotherapy is central to the overall cancer research effort.
But advances in radiation oncology are largely hidden from public view, which is not helping the field to obtain funding. A survey of people in Britain, for example, found that only 10% thought radiotherapy was a “modern, cutting edge treatment” as opposed to 40% for cancer drugs. Only occasionally, such as with a perceived shortage of proton machines, does the latest technology make news; more likely, there will be publicity for a lack of standard radiotherapy care.
In reality radiotherapy has contributed a great deal to progress against cancer over recent decades, not least through major advances in the technology of conventional radiation delivery, with the arrival of systems such as IMRT and IGRT (intensity modulated and image guided radiotherapy), and also arc therapy, that allow higher doses on more precise volumes and spare more normal tissue. New treatment approaches that combine radiation with chemotherapy have now become a standard of care in a number of cancers. And with more advances in molecular biology opening up new avenues to explore, radiation oncology seems particularly lively at present, despite a lack of funding.
Record numbers of abstracts on clinical trials and studies – nearly 2,900 – were presented this September at the conference of the American Society for Radiation Oncology (ASTRO). Europe’s own radiation oncology society, ESTRO, also had a successful conference this year, with more than 5,000 participants, and the radiation oncology group in the EORTC – Europe’s largest collaborative cancer clinical trials organisation – has a number of important collaborative trials running or about to start.
There is broad agreement among radiation oncologists about current clinical standards and areas of research that are ongoing and that show most promise. As with drug development, the aim is to provide more precise and personalised treatment, given radiotherapy’s long history as a ‘one size fits all’ approach and as essentially a single physical technology which can kill cancer cells but which can also severely damage normal tissue. The main research work can be seen in two categories, biology and physics, with extensive overlap between the two.
Precision targeting, photons to ions
Improving the precision of radiotherapy is certainly one of the major branches of medical physics. Amir Abdollahi, head of the Max Eder translational radiation oncology research group at Heidelberg, Germany, points to IMRT as “among the first big steps in precision for targeting tumours”.
“Conformal irradiation of tumours, using up to nine or more irradiation angles, allows for higher doses while sparing critical organs at risk, close to the tumour margin. However, this benefit is bought at a higher volume of normal tissue receiving a lower dose of irradiation,” says Abdollahi. This technique is proving to be especially useful in improving the quality of life in patients with cancers such as head and neck, he says, and with rapid progress in radiotherapy software and hardware cutting the cost and time needed for IMRT, it is increasingly used in many cancer types.
IMRT is essentially a technological advance, as is the use of particles in the form of protons, which Abdollahi describes as “the next step” in applying more precise doses to tumours. Proton therapy is not very different from photon radiation in terms of DNA damage complexity and tumour cell kill, he says. “However normal organs at risk can be much better spared compared to conventional photon irradiation, and also the volume of normal tissue receiving a lower radiation dose can be significantly decreased.”
The latter is of great importance for paediatric oncology, he adds, where secondary cancers could be of concern.
“Now we are working with larger particles at Heidelberg. In addition to the precision of protons, heavier ions such as carbon ions can densely ionise surrounding tissue, generating irreparable DNA damage. Their efficacy may also be less dependent on tumour oxygenation levels, making it easier to eradicate radioresistant hypoxic tumour cells,” says Abdollahi. Other potential radiobiological differences need to be systematically investigated in comparison with proton and conventional radiation, he says.
A wealth of biological ideas
There is a wide spectrum of translational research underway using the different forms of radiation techniques and qualities, and also advanced imaging techniques.
The biological work falls into several camps, says Abdollahi, and includes overcoming hypoxia in tumours; radiosensitisers that enhance the effect of radiation; chemotherapy and targeted drugs that can cooperate with radiation; gene signatures that could predict sensitivity to radiation; agents that offer protection for normal tissue; and novel areas such as angiogenesis and immunotherapy in tumour–stroma communications, where researchers are also looking to ‘modulate’ radiation in various ways.
The complexity of research varies. There are studies of radiation with existing targeted agents, such as inhibitors of the EGFR pathway, to see whether it is still beneficial in combination with particle therapy. “This is a straightforward translational question,” says Abdollahi. More complex is finding causal links, or pathways, that are affected by radiation and using those pathways to sensitise cells to radiation.
Hypoxia
Take hypoxia, which is a long-standing area of study and which has been an obvious phenomenon to attack because it is a common reason for the failure of local treatment. Conventional radiotherapy works by damaging the DNA of dividing tumour cells, mostly through the action of free radicals that are promoted by oxygen. But as solid tumours often restrict oxygen through a poor blood supply, they also develop radio-resistant areas that can be unstable and change in location and time.
Hypoxia can render tumour cells two to three times less susceptible to radiation. A number of approaches over almost 50 years have been tried to overcome it, including hyperbaric oxygen, sensitising drugs such as nimorazole, and hypoxia-activated cytotoxic drugs that target hypoxic cells.
Despite this effort and scores of trials, Jens Overgaard, a European expert in the field at Aarhus University Hospital, Denmark, lamented in 2007 that hypoxic radio-sensitisation is “adored and ignored”. Although “ample data exist to support a high level of evidence for the benefit of hypoxic modification… [it] still has no impact on general clinical practice,” he wrote in the Journal of Clinical Oncology (vol 25, pp 4066–74).
Work of course continues, especially in head and neck cancers, as hypoxia is such an important response predictor. Investigators are using techniques such as PET imaging with tracers to identify hypoxic areas and then target them with radiation ‘dose painting’ (see below). This could help stratify patients into higher risk groups for precise, higher doses. Other researchers are looking at genomic signatures of hypoxia that could identify people most likely to benefit from the radiosensitiser, nimorazole.
As Jacques Bernier, director of radio-oncology at the Genolier Clinic in Switzerland, points out, hypoxia is a key area for the crossover between physics, biology and also computer science: “Radiation dose painting to target intra-tumoural radio-resistance levels is likely to be more and more used thanks to the increasing sophistication of radiation planning and delivery tools,” he says.
Chemoradiation
Radiosensitising drugs are a major research field on their own, as are chemotherapy and targeted anti-cancer agents that can have a synergistic effect with radiotherapy, including acting as radiosensitisers.
There has been considerable success with chemoradiation, as Bernier notes. “Concomitant chemoradiotherapy is now applied in large populations of patients presenting with locally advanced disease in brain, head-and-neck, lung, uterus and various digestive tract malignancies,” he says.
Drug–radiation interactions have now been studied for several decades “in terms of spatial cooperation, cytotoxic enhancement, biological cooperation and temporal modulation,” he notes. An outstanding example for a newer targeted agent – likely to be mentioned by any oncologist – is the combination of radiotherapy with an anti-EFGR drug in head and neck cancers, as EGFR is almost always overexpressed in squamous cell carcinomas in these sites.
“It’s a wonderful example of successful translational research – in a significant number of patients with head and neck carcinoma, the use of bioradiation with cetuximab is nowadays acknowledged as level I evidence by bodies such as ESMO,” says Bernier. (For a paper on milestones in chemoradiation see JCO 2014, 32:1173–79, which also describes how cisplatin became a standard in head and neck cancer.)
Obstacles and slow progress
Despite its clear potential, it is proving hard to find funding to back research into chemoradiation. Abdollahi says there is little support from the major makers of radiotherapy equipment, while drug companies are often reluctant to fund trials that use their products in chemoradiation combinations, as they see little prospect of benefit. This is particularly true where older off-patent agents are used, but even with newer agents, the quantities involved tend to be far smaller than when the same agent is used as a standalone medical therapy.
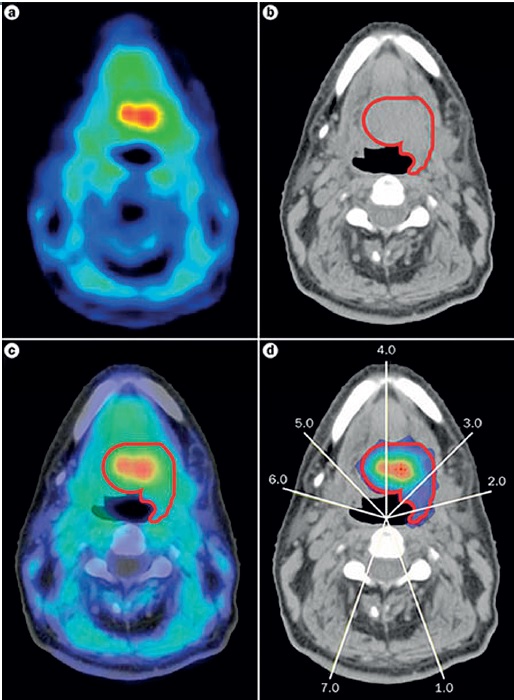
The fact that many agents of interest have only been investigated in patients with advanced cancer presents an added hurdle, says Abdollahi. “Radiotherapy has one of the most attractive populations for investigating combination regimens. In addition to improving local tumour response to irradiation, patients with locally advanced cancer and a poor prognosis (e.g. with lung or pancreatic cancer) may benefit from concurrent and maintenance pharmacological treatments by preventing the formation of distant tumour metastases.” Yet most new compounds are investigated in heavily pretreated end-stage patients who have already developed disseminated metastases, he says, as these populations are larger and therefore of more interest to pharmaceutical companies.
“Drug companies are often reluctant to fund trials that use their products in chemoradiation combinations”
Investigators therefore have to go back to square one and conduct toxicology studies on combinations, as a drug that is of low toxicity on its own may be more toxic when used with radiation. And this is leading to bottlenecks at the preclinical to phase I stages, says Abdollahi. It is ironic, he notes, that new classes of drugs, DNA-repair inhibitors such as PARP, are investigated in phase III trials in combination with chemotherapy rather than radiation, despite the fact that they work in a complementary way.
Commenting though on what we can expect in the future, Bernier says: “It is too early to say what will be the exact place of combination of radiation with targeted therapies, despite the success of anti-EGFR-based bioradiation.”
Philippe Lambin, medical director at the Maastro Clinic, a radiotherapy institute in the Netherlands, adds that another major barrier to the research is effects on normal tissue. “We need preclinical studies on both tumours and normal tissue, as you must get the therapeutic ratio right – in practice you always irradiate normal tissue – but there are few labs in the world that can do this,” he says. “We should have certified labs where we can do these experiments.” Current chemoradiation regimens are often at the limits of toxicity, although additive toxicity in combinations can be avoided by giving drugs and radiation in sequence.
Radiosensitivity of normal tissue varies greatly among patients, so identifying those who can more safely receive higher doses is important. Developing predictors of who will suffer from side-effects from radiation is the subject of a European programme called Requite (see requite.eu) and also the Radiogenomics Consortium, set up in 2009, which has the specific goal of producing assays to predict risk of toxicities after radiation therapy, as outlined in a recent paper, ‘Radiogenomics: radiobiology enters the era of big data and team science’ (Int J Rad Onc 2014, 80:709–713).
“Radiotherapy has one of the most attractive populations for investigating combination regimens”
Lambin is a proponent of decision support systems to aggregate data to help make such predictions (see Cancer World Sept–Oct 2013). While he backs the search for definite biomarkers, he sees biomarker data as part of the mix, mentioning for example work on mitochondrial DNA from saliva in lung cancer models combined with prediction models. He also stresses the field of radiomics, in which imaging data that quantifies differences in tumour intensity (a scale for describing radiodensity in CT scans), together with shape and texture, can have prognostic power (for more on this see Nature Communications 2014, doi:10.1038/ncomms5006).
But progress is slow. Bernier says that biomarkers have been extensively investigated to predict the outcome of almost all solid tumours exposed to radiation alone or radiation combined with systemic treatments. “So far, and in contrast with what has happened in medical oncology, it is clear that their clinical relevance remains disappointing in patients treated with radiation combined with chemotherapy or targeted agents. We must identify more powerful biomolecular markers if we want to intensify the role of personalised medicine in patients treated with radiotherapy.”
There are notable exceptions. Bernier mentions HPV (human papillomavirus) status in oropharyngeal carcinomas, hypoxia levels in cervix cancer, and MGMT methylation status in patients with glioblastoma. The role of HPV is an ongoing research area – those who are HPV positive actually have a better outlook, and a recent study indicates that they may safely receive lower dose radiation after chemotherapy. The identification of patients with a ‘silenced’ MGMT DNA repair gene in glioblastoma has been an important advance in predicting response to treatment with temozolomide and radiation for patients with this high-grade brain tumour.
Latest research
At Heidelberg, Abdollahi and his group are now working on the underlying molecular mechanisms and response predictors of novel therapies, especially for the tumour–stroma ‘microenvironment’, including high-throughput techniques on the genome, proteome and so on, with anti-angiogenesis as a particular focus. “Tumours protect their vasculature from radiation-and chemotherapy-induced damage by releasing pro-angiogenic and pro-survival factors,” he says. Immunotherapy is also a promising area, he adds.
Then there is also the proton and carbon ion work with the Heidelberg Ion-Beam Therapy Centre – overall, this is one of Germany’s largest cancer research centres, with a substantial fraction of patients with head and neck cancers and gliomas, for instance, being treated in multimodal trials.
Certainly, genomic work is a promising area, as it is generally in oncology, and many groups are investigating predictive signatures and associations between radiosensitivity and genetic alterations. One group may have taken a lead – Javier Torres-Roca and team at the Moffitt Cancer Center in Florida has developed a signature that can predict radiosensitivity and, importantly, has been validated in breast, rectal and other cancers. Such work is not necessarily welcomed by some in the radiotherapy community who are paid for each treatment episode, as signatures can show who may avoid or receive less radiation.
“Genomic signatures can show who may avoid or receive less radiation”
Meanwhile, Lambin is not waiting for the big drug companies to come up with new agents. A bio-tech start-up from his clinic in Maastricht, DualTPharma, is developing a ‘smart dual drug’ that exploits the overproduction of acid in tumours. The drug targets cells with an inhibitor for a protein that regulates acid (called CA IX), thereby promoting more acid, which could kill cancer cells, and also deploys a radiosensitiser to guide a radiation ‘warhead’ to the target.
Lambin also mentions immunotherapy combined with radiotherapy as one of the most important ways forward, suggesting it could extend treatment from locally advanced to the metastatic stages. When radiation causes cell death it also releases antigens that stimulate the immune system, which can be enhanced by drugs such as IL2 (interleukin 2), which is a class of drug known as a cytokine, but on its own is uncontrolled in the body and can have bad side-effects.
As an example of how to develop this type of immune response, Lambin and colleagues have used an immunocytokine consisting of IL2 coupled to an antibody that binds the combination to tumour vasculature to release cytotoxic T-cells, and they have shown a 75% cure rate in certain animal models. A phase I study has been approved, which will investigate patients with a low number of small metastases (fewer than five, a status known oligometastasis), in which they will receive high-dose radiotherapy and the combination drug, with the eventual aim of seeing if more metastases can be prevented. A study on radiation alone has already been carried out (J Thorac Oncol 2012, 7:1547–55).
Lambin says that oligometastasis is also proving to be an important condition just for treatment with stereotactic radiotherapy (which uses numerous precisely targeted beams). In fact a recent study (Lancet Oncol 2014, 15:387–395) on brain metastases suggests that treating up to ten sites may be at least as good as treating patients with two metastases, and probably better than whole-brain radiation – and that adding targeted therapy could be the next step.
Abdollahi also points out that the major differences in tumour type can influence the design of radiotherapy research. Much of the emphasis is on improving local control, where radiation has had much success in, say, breast and rectal cancer. However, aiming for similar local control, e.g. using dose escalation, in tumours where there is a high failure rate within two to three years, such as in glioblastoma or lung cancer, may not be appropriate.
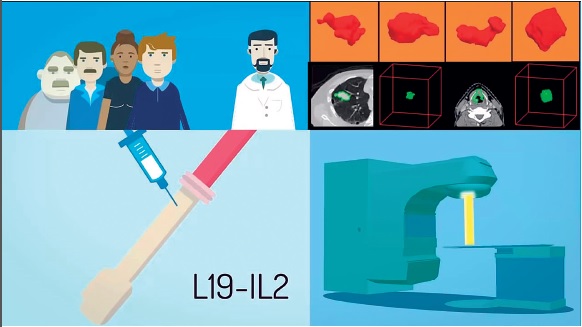
That new approaches could be quite simple is also shown by yet another trial Lambin is involved with, which is using nitroglycerin to address hypoxia when treating non-small-cell lung cancer. Nitroglycerin improves blood flow but has not been studied with radiotherapy – so in a phase II trial 60 patients have been given a nitroglycerin patch during a radiotherapy course, with the effect on blood supply measured by dual energy CT and HX4 (a blood hypoxia imaging marker) PET scans.
Bernier is more down to earth about current prospects and considers much of the work is only just beginning. “The tumour micro-environment is an appealing target but we have no clear demonstration so far that it will have a clinical impact in the medium term. How to by-pass radio-resistance in cancer stem cells is an interesting domain that we should undoubtedly explore more extensively.
“Immunology remains practically unexplored for its impact on radiation modulation, except in patients with non-Hodgkin’s lymphoma who are benefiting now from radio-immunotherapy. And research on the radioprotection of normal tissues can still be considered in its infancy, as most attempts to deliver drugs to tissue next to the tumour and exposed to radiation have yielded inconclusive results.”
The top three subjects for grants listed by an ASTRO task force reporting on biological science in radiotherapy as of November 2012 were tumour microenvironment, normal tissue and radiosensitisers, but despite all the promising work in these areas, little has made it into clinical practice (Int J Radiation Oncol Biol Phys 2014, 88:11e17).
“How to bypass radio-resistance in cancer stem cells is an interesting domain that we should explore more”
Funding and organisation
In short, more work needs to be done before these sorts of novel approaches have a chance to make their way into the clinic. The question is where the capacity and funding to carry out that work will come from.
The ASTRO task force concedes that “radiation oncology is a relatively small specialty with a limited number of committed investigators and finite resources,” while a paper on funding suggests that “the field of radiation oncology is underfunded by the NIH [US National Institutes of Health] and that the current level of support does not match the relevance of radiation oncology for cancer patients or the potential of its academic workforce” (Int J Radiat Oncol Biol Phys 2013, 86:234–240).
In fact, in fiscal year 2013, this paper reckons that radiation oncology research in the US received only 1.6% ($85.5 million) of the $5.4 billion in cancer research funding from the NIH, although as in Europe, it is not easy to measure exactly where funds are going and what all the sources are, given the diversity of the field, which takes in sectors such as medical physics and radiobiology.
A paper published last year in Science Translational Medicine (5:173sr2), on ‘new paradigms and future challenges in radiation oncology’, adds that “it is critical to widen the therapeutic window for radiotherapy at the biological level, particularly in situations where the physical and technical advances could be nearing a plateau… but we are left with the impression that there are many uncoordinated and competing research efforts.”
Wider cooperation between groups would seem to be part of the answer. Lambin says Maastro is one of the European centres to have research tie-ups with several top North American groups such as Moffitt, Dana-Farber and UPenn. Heidelberg is part of a consortium with Tufts and Harvard, and Abdollahi says an important collaboration is about to start between the US National Cancer Institute and Germany’s National Centre for Radiation Research in Oncology (NCRO), which combines the centres in Heidelberg and Dresden, to focus on translational research.
A paper published last year, ‘Lessons learned from radiation oncology clinical trials’, suggests that an international consortium may be needed to speed radiation modifiers into clinical use, as at present they are just too much of a “secondary path, spin-off, or occasional afterthought to drug development” (Clin Cancer Res 19:6089–6100). The Radiogenomics Consortium, as noted above, could be a model. It currently has more than 170 members from 90 institutions in 20 countries, but is making no promises yet.
“An international consortium may be needed to speed radiation modifiers into clinical use”
There are some other encouraging signs of serious national investment in the sector. Sweden, for example last year announced a ‘national test bed for innovative radiotherapy’ via university hospital and industry collaboration. In the UK, the Radiotherapy–Drug Combinations Consortium (RaDCom) is another recent initiative. But generally Europe has a shortage of centres of excellence in oncology.
Given the high proportion of patients treated with radiotherapy, and the variety of promising areas of research being pursued, it’s not surprising then that many leaders in the field feel their potential contribution to improving outcomes for cancer patients merits more than the estimated 1.6% of cancer research funds coming from the main US funding body.
Finding ways to get greater visibility for the many innovations in the field, and their implications for patients, will be important in winning the argument for the support they need.
Leave a Reply