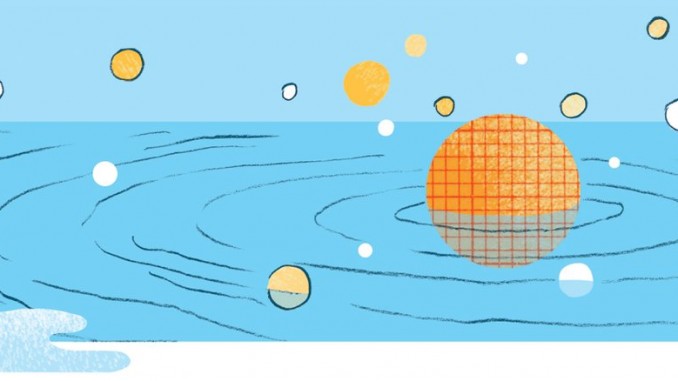
Over the past decade, genetic testing for rare inherited mutations, such as BRCA1 and BRCA2 mutations, has been successfully incorporated into clinical practice. Next-generation sequencing of cancer-susceptibility genes and entire tumour genomes has transformed cancer care and prevention. The discoveries of new cancer syndromes have raised exciting opportunities and potential liabilities for cancer-care providers seeking to incorporate genomic approaches into preventive oncology practice.
This article was first published in Nature Reviews Clinical Oncology vol. 11, no. 11, and is republished with permission © 2014 Nature Publishing Group.
The past decade has witnessed the incorporation of genetic testing for cancer-susceptibility syndromes into the evidence-based practice of oncology, and the emergence of ‘next-generation’ genome scans for cancer-risk loci. Herein, I discuss a series of seminal papers published over the past decade that described new cancer syndromes, but also raised new challenges related to informed consent, incidental findings, and the management of genetic variants of unknown significance or unproven clinical actionability.
In the 1980s and 1990s, rare but highly-penetrant cancer-predisposition genes were identified by studying cancer-prone families that demonstrate Mendelian inheritance of cancer susceptibility. These studies implicated genes, such as BRCA1 and BRCA2, the DNA-mismatch-repair genes (relevant for colon cancer), TP53 in Li–Fraumeni syndrome, and APC in familial adenomatous polyposis. The genetic basis of these and other syndromes had a powerful impact on the practice of preventive oncology. The incorporation of genetic testing for BRCA mutations in breast cancer marked one of the first applications of ‘personalised’ genomics in medicine, and enabled ‘targeted’ cancer screening, prevention and, in some cases, the ability to personalise therapies according to the patient’s genetic lansdcape.[1] The translation of BRCA testing to clinical practice was highlighted by Domchek and colleagues[2] who showed that preventive surgery of the ovaries over a 34-year period decreased mortality in a cohort of 2,482 women with BRCA1 or BRCA2 mutations; compared with women who did not have salpingo-oophorectomy, women who underwent this procedure had a 60% decrease in all-cause death rates, driven by lower mortality associated with both breast and ovarian cancer.[2] In this study, the subset of women found to have occult microscopic ovarian cancer at the time of ‘preventive’ surgery were excluded from analysis.[2] During subsequent years, risk-reducing ovarian surgery, along with breast MRI, the option of prophylactic breast surgery, and hormonal chemoprevention, became standard practice in preventive oncology.[1]
In the past decade it had become obvious that highly penetrant cancer genes (such as BRCA1/2 and MSH2) did not account for the bulk of familial risk of the common hereditary cancers. A debate ensued regarding whether there were many common low-risk genetic variants or undiscovered rare high-risk variants, which would explain the ‘missing heritability’ of cancer. A pivotal paper tested the ‘common variant’ hypothesis using the emerging technology of ‘gene chips’ to assess hundreds of thousands of single nucleotide polymorphisms (SNPs).[3] In a two-stage design, 227,876 SNPs were assessed in 4,398 breast-cancer cases and 4,316 controls, identifying 30 SNPs of interest, which were further analysed in 21,860 cases and 22,578 controls.[3] The SNP that emerged as the best ‘hit’, which was proximal to the gene FGFR2, had a relative risk of around 1.2-times the baseline risk, compared with BRCA1, which elevated risk of early onset breast cancer by up to 40-fold.[3] Subsequent genome-wide association studies of other cancer types identified hundreds of hits near potentially causal genes, which were all statistically significant, but none of a magnitude to influence preventive management in the clinic.[4] A possible exception to this lack of clinical utility emerged from studies we performed as part of an international consortium investigating modifiers of risk in the carriers of BRCA mutations. In studies involving tens of thousands of BRCA1/2 mutation carriers worldwide, panels of risk-associated SNPs could partition breast cancer risk from 20% up to 100% in BRCA-mutation carriers.[5] These findings will likely mark the first application of SNP-based risk profiling to inform clinical management of individuals with hereditary risk of a common cancer.
Over the second half of the past decade, a shift to identifying rare genomic variants was made possible by the emergence of next-generation sequencing (NGS) approaches. NGS involves a series of repeating sequencing reactions, performed and detected automatically, with the production of thousands to millions of simultaneous sequence reads. An immediate and obvious application of NGS was to sequence several genes at the same time. A technological tour de force prefigured the current era in ‘cancer panel’ testing. Using targeted capture and massively parallel genomic sequencing, a group at the University of Washington screened 21 candidate genes in 360 women with ovarian cancer.[6] Strikingly, 24% of these women carried germline loss-of-function mutations in genes such as BRCA1, BRCA2, BARD1, BRIP1, CHEK2, MRE11A, MSH6, NBN, PALB2, RAD50, RAD51C, and TP53.6 Fuelled by this technological innovation, plus the equally impactful loss of patent protection for BRCA1 and BRCA2 sequence analysis, a plethora of commercial cancer panels flooded the oncology marketplace.
At the same time, NGS technologies were rapidly applied to studying unexplained familial cancer clusters. Over the past five years, whole-exome sequencing (WES) and whole-genome sequencing (WGS) has resulted in a renaissance in the discovery of new syndromes of cancer susceptibility.
Cancer susceptibility syndromes*
Familial pancreatic cancer
PALB2 identi ed by exome sequencing; ATM identi ed by exome sequencing and WGS
Familial ovarian cancer
BRIP1 identi ed by WGS
Familial pheochromocytoma
MAX identi ed through exome sequencing
Acute myelogenous leukaemia (with Emberger syndrome)
GATA2 identi ed by exome sequencing
Familial Hodgkin lymphoma
NPAT identi ed by exome sequencing
Familial pre-B-cell acute lymphoblastic leukaemia
PAX5 identi ed by exome sequencing
Familial melanoma
MITF identi ed by WGS; TERT identi ed by targeted sequencing
Familial mesothelioma, melanoma and renal-cell cancer
BAP1 identi ed through exome and targeted sequencing
Hereditary mixed polyposis syndrome (HMPS)
GREM1 identi ed by targeted sequencing
Colorectal adenomas and colon cancer
POLE and POLD1 identi ed by WGS
Familial breast cancer
XRCC2 and FAN1 identi ed by exome sequencing; PPM1D (mosaic) by targeted sequencing
*Discovered recently by next-generation sequencing4
WGS – whole-genome sequencing
One of the early applications of this technology came from a group at the Johns Hopkins University, who applied WES of 20,661 coding genes in a single case of familial pancreatic cancer.[7] Of 15,461 germline variants not found in the reference human genome, a deletion of four base pairs within the PALB2 gene was discovered and tested as a pancreatic-cancer-susceptibility gene.[7] Despite this early report, we and others have failed to confirm PALB2 as a major factor in hereditary breast–pancreas-cancer families; however, PALB2 maintained its status as a rare breast-cancer-susceptibility gene.
Another example of a new syndrome with a striking phenotype was described by Testa and colleagues in 2011,[8] on the basis of their observation of gene clustering of mesotheliomas and melanomas. Using exome sequencing strategies, germline mutations were discovered in the gene encoding BRCA1-associated protein-1 (BAP1) in two families with multiple cases of mesothelioma, and in some cases of uveal melanoma.[8] These findings built on the earlier observation of inherited germline BAP1 mutations in uveal and cutaneous melanocytic tumours. Remarkably, this syndrome was extended by other groups to include renal-cell cancers in rare families.
In some cases the ‘new’ familial cancer types studied were not rare. For example, we studied families with acute lymphoblastic leukaemia, the most-common malignancy of childhood, and identified a mutation in a lymphoid-associated transcription factor, PAX5, in two such families,[9] with a third Israeli family more recently reported to harbour the same mutation. These ‘new’ cancer syndromes have redefined our notion of inherited cancer (see box).
Oncologists will soon be screening
the inherited genomes of all patients with cancer
Despite these advances over the past decade, clinical interventions for these syndromes remain relatively rudimentary, and the ethical implications of these discoveries remain daunting. Risk reduction for the adult-cancer syndromes includes organ removal surgeries.[1] True genetic prevention using assisted reproductive technologies is an option oncologists should remember to discuss with their younger patients, or patient’s families, taking into account ethical or religious considerations. A broader ethical debate has emerged regarding the extent to which incidental, or secondary genetic findings, termed the ‘incidentalome’, should be disclosed to patients. Particularly challenging for oncologists are the unexpected results of NGS analysis of tumour and normal pairs, which might include identification of genetic predispositions to non-cancer-related diseases, such as cardiac or neurological diseases.[10] A vigorous discussion is in progress regarding the potential obligations of physicians to inform individuals of incidental genetic findings.
At the same time there have been recent calls for population-based screening, ‒ for example BRCA testing of all 30-year-old women worldwide. Although such requests by laboratory-based scientists have the best intentions, they overlook a more-pressing clinical reality: oncologists will soon be screening the inherited genomes of all patients with cancer. In both scenarios, population testing of healthy individuals and tumour–normal screening in patients with tumours, we must recognise what has been learnt over the past decade: not all individuals wish to know all genomic information; risks might reflect both population heterogeneity and differences in penetrance; and not all genomic information is clinically actionable. Oncology has become the ‘ground zero’ for a tectonic shift in paradigms regarding personalised medicine, both for targeted treatment as well as prevention based on genomic profiles.
References
1. FJ Couch, KL Nathanson and K Offit. (2014) Two decades after BRCA: setting paradigms in personalized cancer care and prevention. Science 343:1466–70
2. SM Domchek et al. (2010) Association of risk-reducing surgery in BRCA1 or BRCA2 mutation carriers with cancer risk and mortality. JAMA 304:967–975
3. DF Easton et al. (2007) Genome-wide association study identifies novel breast cancer susceptibility loci. Nature 447:1087–93
4. K Stadler, KA Schrader, J Vijai et al. (2014) Cancer genomics and inherited risk. JCO 32:687–698
5. MM Gaudet et al. (2013) Identification of a BRCA2-specific modifier locus at 6p24 related to breast cancer risk. PLoS Genet 9:e1003173
6. T Walsh et al. (2011) Mutations in 12 genes for inherited ovarian, fallopian tube, and peritoneal carcinoma identified by massively parallel sequencing. Proc Natl Acad Sci USA 108:18032–37
7. S Jones et al. (2009) Exomic sequencing identifies PALB2 as a pancreatic cancer susceptibility gene. Science 324:217
8. JR Testa et al. (2011) Germline BAP1 mutations predispose to malignant mesothelioma. Nat Genet 43:1022–25
9. S Shah et al. (2013) A recurrent germline PAX5 mutation confers susceptibility to pre-B cell acute lymphoblastic leukemia. Nat Genet 45:1226–31
10. Y Bombard, M Robson and K Offit. (2013) Revealing the incidentalome when targeting the tumor genome. JAMA 310:795–796
Acknowledgements
The author would like to acknowledge funding support from The Robert and Kate Niehaus Clinical Cancer Genetics Research Initiative, and The Breast Cancer Research Foundation
Affiliations
Department of Medicine, Clinical Genetics Service, Program in Cancer Biology and Genetics, Sloan-Kettering Institute, Memorial Sloan Kettering Cancer Center, and Department of Medicine and Public Health, Weill Cornell Medical College, Cornell University, New York, New York
Leave a Reply